Attention-Deficit Hyperactivity Disorder in children [ADHD; see e.g. 1 for a review] is a neurodevelopmental disorder characterized by attentional problems, hyperkinesis, restlessness, disturbances in timing. It affects mostly male children 1 with a high genetic component as demonstrated by family studies, and the allelic polymorphism of the dopamine (DA) D-4 receptor gene and the DA transporter protein (DAT) 2. Some of the behavioral problems of ADHD as well as SHR have been linked to dysfunction of the mesolimbic dopamine (DA) system which innervates the nucleus accumbens, and the prefrontal cortex [see 3 for a rev.] and is a major component of a multiple system involved in attentional and reinforcement mechanisms 4,5. DA is known to bind to a heterogeneous class of synaptic and extra-synaptic receptors of the D-1 and D-2 subfamilies [see e.g. 6, and refs. within], thus increasing the signal-to-noise ratio 7.
We have focused our attention on the most anterior portion of the forebrain including structures such as the nucleus accumbens (ACB). The ACB constitutes an anatomical and physiological unit, which has been divided into three subterritories, the "rostral pole", the "core", and the "shell" 8. It is the target of a wide glutamate innervation from prefrontal cortex, midline intrathalamic nuclei, hippocampus, basal amygdala 9,10, as well as an extensive DA projection from the ventral tegmental area (VTA). In turn, it sends efferent to all mesencephalic DA sources 11. The "core" projects to the prefrontal cortex, while the shell and rostral pole have a wide output to the lateral hypothalamus, basal amygdala and hippocampus 11.
Therefore, using the D-1 and D-2 dopamine receptor types, CaMKII, and the transcription factors c-fos and JUN-B as a marker of neuronal activity, the aim of this study was to investigate its spatial distribution in the antero-posterior plane in the SHR and WKY controls
Materials and Methods
Animals:
Juvenile (4-wk-old) male Spontaneously Hypertensive rats [SHR; n= 6) and Normotensive Wistar-Kyoto controls (WKY; n= 6) (Charles-River, Italy) were housed in standard cages during a two-week period for acclimatization and handling.
Procedures :
Rats were either given a daily intraperitoneal injection of vehicle (controls) or methylphenidate (3 mg/kg) for 15 days. rats were sacrificed either 1 day or 3 days after the last injection.
Receptor binding assays:
The assays were carried out according to the procedure described earlier 16. A saturation analysis (maximal binding capacity and affinity) was performed using 7 concentrations of the radioactive DA antagonist [3H]-SCH 23390 (Spec. Act. 60-87 Ci/mM; 0.1 - 5.0 nM). D-1 excess unlabelled antagonist SCH 23390 was used, to control for non-specific binding. For D-2 receptor subfamily two competition studies were carried out using 4.2 nM 3H-raclopride and 5 nM 3H-quinpirole with unlabelled spiperone (0.1 nM - 1 mM) and 7-OH-DPAT (0.1 nM - 10 mM) as unlabelled displacers. In both series of binding assays, ketanserin (1 mM) was added to resolve the DA and serotonin components of the binding 17.
Autoradiography:
The entire anterior forebrain was serially sectioned and randomly assigned to different experiments. The slides with tissue sections were incubated using optimized conditions and exposed to [3H]-sensitive films (Amersham) for 18 days for SCH 23390, 8 weeks for raclopride and 3 months for quinpirole. Films were developed and optical densities (OD) of sections were analyzed with reference to co-exposed standard [3H]-microscale (calibration curve to convert OD to receptor concentration). The autoradiograms were taken by a CCD camera (Hamamatsu Photonics, Italia), and converted to a 640 x 480 pixel file by an acquisition board and a computer-assisted image analysis system (MCID-M4; Imaging Res. Inc, Canada). The recognition of forebrain structures was accomplished by inspection of Nissl-stained adjacent sections and the Paxinos and Watson atlas of the rat brain 18.
Antibodies:
The primary antibody was a mouse monoclonal antibody towards the alpha-subunit of the CaMKII (Boehringer, Mannheim) or a mouse polyclonal antibody against c-fos and JUN-B For transcription factors the corresponding control peptides were used to test specificity. For both markers the secondary antibody was a goat anti-mouse serum and the revealing system was the avidin-biotin complex (ABC; Vector Laboratories, Burlingame, CA).
Perfusion:
At the age of 6 wks, which corresponds to the pre-hypertensive state for SHR, rats were deeply anesthetized by continuous exposure to ether and perfused transcardially with physiological saline followed by 4 % paraformaldehyde in 0.1 M phosphate buffer (PB, pH 7.3). Brains were postfixed in the same fixative for 2 h, washed in phosphate-buffered saline (PBS), soaked in 30 % sucrose for cryoprotection, frozen in isopentane on dry ice and stored at –80 °C.
Immunocytochemistry:
Fifty mm-thick cryostat coronal sections were collected in ice-cold PBS containing 0.1 % sodium azide. Sections were free-floating immunostained for the detection of the alpha-subunit of CaMKII [see ref. 19] or the transcription factors cited above, as previously described 19-22. The dilution of the antibody was 1:500. The sections were exposed to diaminobenzidine (DAB, 100 mg in 200 ml buffer), and a Nickel-Cobalt intensification procedure was performed. To assess the specificity of labeling, control experiments were performed by incubating overnight the primary antibody with a ten-fold excess of peptide antigen in PBS. The sections were mounted on poly-L-lysine coated slides and coverslipped. Images were taken by a CCD camera (Hamamatsu Photonics Italia) mounted on a Zeiss Axioskop microscope and analyzed quantitatively with an image analysis system (MCID-M4; Imaging Research Inc., Canada).
Data acquisition and analysis:
The autoradiograms were taken by a CCD camera (Hamamatsu Photonics, Italia), and converted to a 640 x 480 pixel file by an acquisition board and a computer-assisted image analysis system (MCID-M4; Imaging Res. Inc, Canada). The recognition of forebrain structures was accomplished by inspection of Nissl-stained adjacent sections and the Paxinos and Watson atlas of the rat brain 18. Saturation and competition analyses were carried out by conventional algorythmses.
Images from icc-treated sections under light-microscope (Zeiss Axioskop) were taken by ccd camera (Hamamatsu Photonics Italia) and analyzed quantitatively with an image analysis system (MCID-M4; Imaging Research Inc., Canada). The ACB was outlined for each section and analyzed according to Nissl-stained adjacent sections. The pole was defined as the rostral portion of the ACB to its fusion with striatum. The core was divided from the shell of the ACB throughout the posterior horn of the anterior commissure. Immunoreactive elements, i.e. mainly somata and dendrites, were considered positive when they fell into the range between 0 and 140/160 (gray levels 0-255). The proportional area covered by immunoreactive elements was analyzed bilaterally by planned comparisons within area by two-tailed t-test for unpaired data. The rejection level was set at p < 0.05.
Data were expressed mean ± s.e.m. and submitted to 3-way analysis of variance line x treatment x rostro-caudal level (Anova).Between-line planned comparisons were made by two-tailed t-test for unpaired data. Left-to-right differences were analyzed by t-test for paired data. The rejection level was set at p ³ 0.05.
Back to the top.
Results
1. DOPAMINE RECEPTOR AUTORADIOGRAPHY
Computer-assisted high resolution image analysis demonstrated in the shr a higher density of DA D-1, but not D-2, receptors (Carey et al., in press).
This was demonstrated by saturation analysis in a pool of sections from anterior but not posterior portions of different forebrain structures. A significant higher level of D-1 receptors was found in the core and shell of the accumbens (fig. 1A), the caudate-putamen complex (fig. 1C, upper panel under basal conditions) and the olfactory tubercle (fig. 1D, upper panel under basal conditions)
A subchronic treatment with methylphenidate (3 mg/kg, i.p. , MP ) daily for 15 days did not have any effect in the anterior core and shell of the accumbens (fig. 1B) and in the olfactory tubercle (fig. 1D, ), but it down-regulated it in the caudate-putamen (fig. 1C, lower panel)
There was not significance differences in two families between Shr and Wky under basal conditions nor following MP treatment, as assessed by competition analyses using quinpirole and raclopride as radioactive ligands and spiperone and 7OH-DPAT as not labeled displacers (data not shown).
2. Ca2+/CALMODULIN-DEPENDENT PROTEIN KINASE II
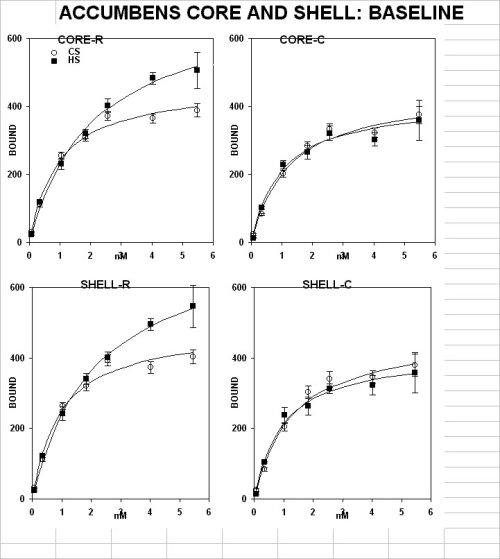
Figure 1a
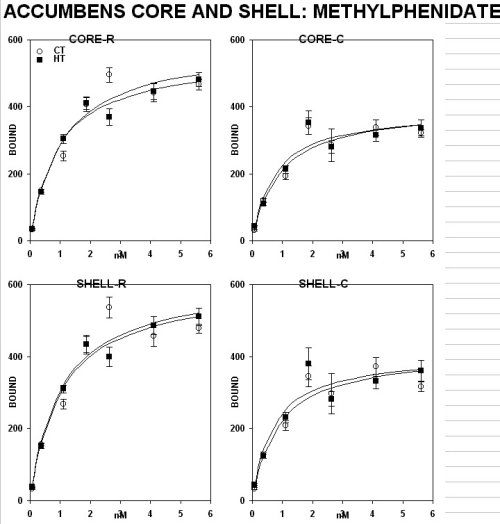
Figure 1b
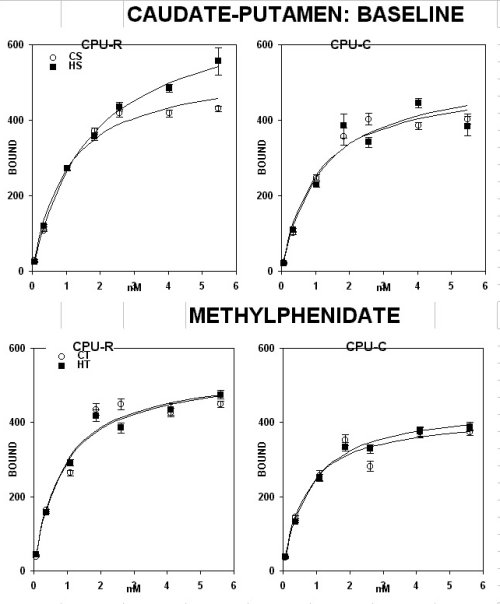
Figure 1c
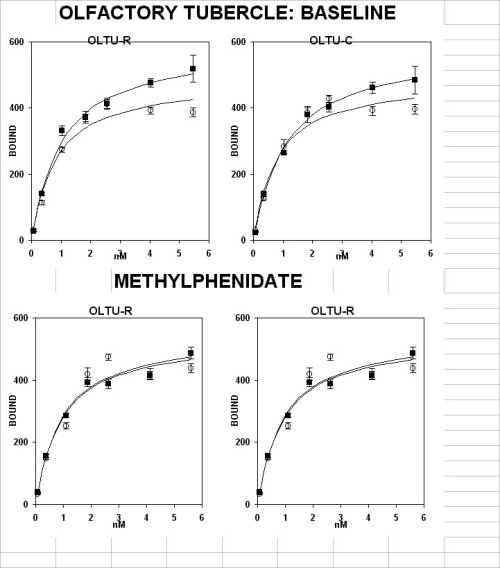
Figure 1d
The Shr showed a reduced number of elements positive for CaMKII in the pole and shell but not in the core in n. accumbens under basal conditions (fig 2, upper panel).
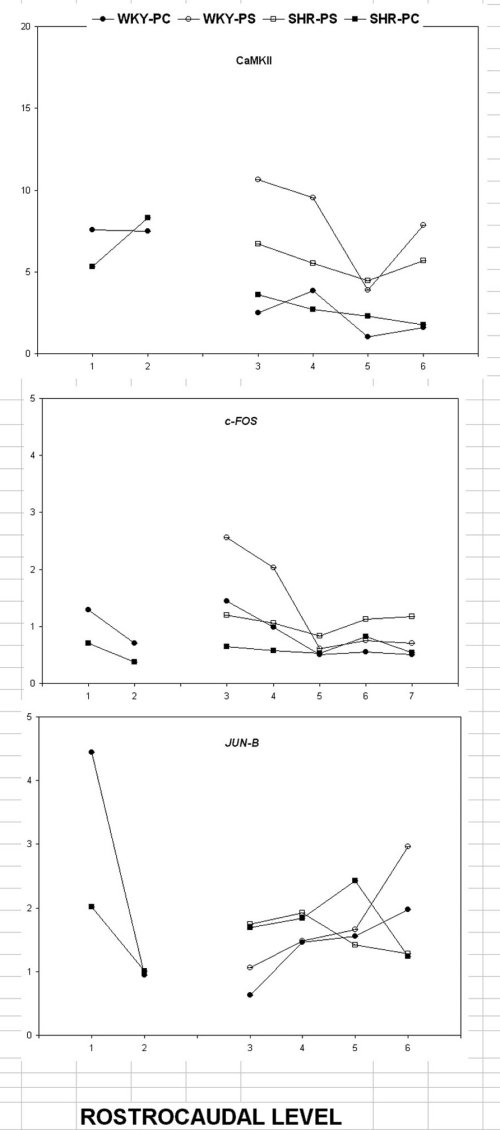
Figure 2
This was restricted to the most anterior portions of the forebrain.
In addition, subchronic treatment with MP reversed the CaMKII across the forebrain (data not shown).
3. TRANSCRIPTION FACTORS
The Shr showed also a reduced expression of the transcription factor c-FOS in the shell but not the core of n. accumbens (fig 2, middle panel).
The rostral caudal-pattern was similar to the one described above for CamKII, being restricted to the most rostral portions of this structures.
In contrast, JUN-b expression (fig2 ,lower panel) demonstrated a rostro-caudal pattern which was the opposite of the one described for c-FOS.
In fact, there was a lower expression in the anterior portion as compared to the posterior ones.
Subchronic MP-treatment increased the expression level of the C-FOS in the Shr to the level of the Wky controls
across the forebrain with the exception of the anterior portions of the accumbens core and shell, the frontal cortex, and the ventral pallidum (data not shown).
Moreover, no effect was observed in the central and medial amygdala nuclei (data not shown).
Back to the top.
Discussion and Conclusion
There appears to be a discrete defective segment in the anterior forebrain of the shr model of adhd that is comprised between +2.70 and 1.70 mm anterior to the bregma.
This has been demonstrated by multiple neuronal markers, namely (1) an increased density of dopamine d-1 (but not d-2) receptor binding sites, (2) a reduced expression level of CamKII and (3) transcription factors.
This defect is partially reversed by subchronic treatment with an inhibitor of the re-uptake of biogenic amines, methylphenidate (mp; Ritalin), which shows differential effects. in fact, this drug reduced the density of DA D-1 binding sites in the anterior caudate-putamen complex, but not in the accumbens core and shell.
MP reversed the expression level of CaMKII across the forebrain with the sole exception of the central amygdala nucleus.
Interestingly, MP increased the expression level of the protein c-fos across the forebrain with the exception of the anterior portions of the frontal cortex, the n. accumbens core and shell and the ventral pallidum.
Intriguingly, this was observed also in the central and medial amygdala nuclei. The latter discrete effect limited to two amygdala nuclei might be interpreted as secondary to the effect in the accumbens shell with which it is connected.
This segmental defect, including a discrete portion of the anterior forebrain, affects strategic sites for the control of executive functions, attention, motivation and reward processes, the motor output from the ventral pallidum and the thalamus, and part of the amygdala. Therefore, it might explain the clinical disturbances of ADHD children such as hyperactivity, inappropriate movements, low attentive levels, and the emotional problems which are associated with adhd in a large percent of adhd cases.
This segmental defect, which might reflect a deranged "prosomeric" organitation23, of the Shr forebrain, could be temporary, due to a delayed development, and result in an inappropriate matching at neural interfaces such as nucleus accumbens, caudate-putamen and frontal cortex.
Alternatively, this defect might be permanent. In the latter case, functional imaging studies in children should be carried out to validate the importance of these findings from the animal model.
At the same time, the organization of the anterior forebrain during development should be investigated.
The finding of defective transcription factors, which are normally involved in the organization of the anterior forebrain, may lead to the development of biological markers and to a population screening by simple blood sampling.
This would enormously alleviate the suffer-ance to the individuals affected by ADHD and their family, and reduce the high costs to the society.
The higher density of DA D-1 binding sites is of great interest since is not associated to similar changes in D-1 but not D-2. the differential distribution of the two DA families validates the specificity of the defect.
On the one hand, the differential response to mp-treatment in the caudate-putamen complex but not in the accumbens and olfactory tubercle in Shr might explain the positive effect of MP observed in children on the hyperactivity but not attention deficit symptoms.
on the other hand the unbalance between D1 e D2 rec. families should pivot positron emission tomography studies in vivo in children using specific d1 receptor ligands. Further the unbalance is likely to be corrected by specific treatment with D1 rec. ligands. In fact, increased density of D-1 binding sites is coherent with a reduced DA release and a reduced number of accumbal elements and modules available for limbic-motor integration in a discrete segment of the anterior forebrain.
However, the possibility that higher D1 binding sites reflect an increased number non functional receptors e.g. due to delayed "pruning" 24 should be investigated.
The emerging evidence of a segmental defect in the development of the Shr brain might be due to (i) selective degeneration of DA neurons in the ventral tegmental area, (ii) a delayed development of the affected segment, or (iii) a disintegration between local factors and environmental signals.
In addition, this defect is partially reversed by environmental stimulation (in preparation), thus demonstrating an interactive role of the environment with genetic components.
Therefore, this animal model might reveal putative substrates of the altered attention and reinforcement mechanisms of the Shr and ADHD children, and should pivot functional imaging studies in ADHD children and search for biological markers for an early diagnosis of the syndrome.
SUMMARY
Molecular biology and light-microscope imaging techniques are be used to map in the anterior forebrain of animal model of ADHD, the juvenile pre-hypertensive male SHR and WISTAR-KYOTO (WKY) controls, the spatial distribution of markers of neuronal activity such as (I) Dopamine (DA) D-1 and D-2 receptors by quantitative Autoradiography, (II) The CA2+/Calmodulin-dependent protein kinase II (CamKII), (III) and transcription regulators, such as C-FOS, JUN-B ,by Immunocytochemistry: he results indicates a discrete segmental defect including the most rostral portions of n. accumbens, caudate-putamen and olfactory tubercle, as revealed by higher density of D1 but not D2 dopamine binding sites and reduced expression of CaMKII and C-FOS. The hypothesized segmental defect may explain the hyperactivity and attention deficit in Shr as ADHD children.
Back to the top.
References
This project has been supported EU HCM grant and by Telethon-Italy grant E.513.
1. Gunning, W. B. & Sergeant, J. A. State Art 1, 4-15 (1994).
2. LaHoste, G. J., Swanson, J. M., Wigal, S. B., et al. Molec. Psychiatry 1, 121-124 (1996).
3. Russell, V. A., de Villiers, A. S., Sagvolden, T., Lamm, M. C. L. & Taljaard, J. J. F. Brain Res. 676, 343-351 (1995).
4. Robbins, T. W. & Everitt, B. J. in The Cognitive Neurosciences (ed Gazzaniga, M.S.) 703-720 ( The MIT Press, Cambridge, MA, 1994).
5. Apicella, P., Ljungberg, T., Scarnati, E. & Schultz, W. Exp. Brain Res. 85, 491-500 (1991).
6. Hersch, S. M., Ciliax, D. J., Gutekunst, C.-A., et al. J. Neurosci. 15, 5222-5237 (1995).
7. Servan Schreiber, D., Printz, H. & Cohen, J. D. Science 249, 892-895 (1990).
8. Zahm, D. S. & Brog, J. S. Neuroscience 50, 751-767 (1992).
9. McGeorge, A. J. & Fuall, R. L. M. Neuroscience 29, 503-537 (1989).
10. Kelley, A. E. & Domesick, V. B. Neuroscience 7, 2321-2335 (1982).
11. Groenewegen, H. J. & Russchen, F. T. J. Comp. Neurol. 223, 347-367 (1984).
12. Schulman, H. Curr. Opin. Cell Biol. 5, 247-253 (1993).
13. Kelly, P. T., McGuinness, T. L. & Greengard, P. Proc.Natl.Acad.Sci.USA 81, 945-949 (1984).
14. Morgan, J. I. & Curran, T. Annu. Rev. Neurosci. 14, 421-451 (1991).
15. Sheng, M. & Greenberg, M. E. Neuron 4, 477-485 (1990).
16. Carey, M. P., Diewald, L., Papa, M., et al. Behav. Brain Res. 94, 173-185 (1998).
17. List, S. J. & Seeman, P. Proc. Natl. Acad. Sci. U. S. A. 78, 2620-2624 (1981).
18. Paxinos, G. & Watson, C. The Rat Brain in Stereotaxic Coordinates ( Academic Press, London, 1986).
19. Papa, M., Sagvolden, T., Sergeant, J. A. & Sadile, A. G. Neuroreport. 7, 3017-3020 (1996).
20. Papa, M., Pellicano, M. P., Welzl, H. & Sadile, A. G. Brain Res. Bull. 32, 509-515 (1993).
21. Papa, M., Pellicano, M. P., Cerbone, A., et al. Brain Res. Bull. 37(2), 111-118 (1995).
22. Papa, M., Sergeant, J. A. & Sadile, A. G. Synapse (1996).
23. Rubenstein, J. L., Martinez, S., Shimamura, K. & Puelles, L. Science 266, 578-580 (1994).
24. Andersen, S., Rutstein, M., Benzo, J., Hosteter, J. & Teicher, M. Neurorep. 8, 1495-1498 (1997).
Back to the top.
|