The structural diameter of arteries is modulated by chronic changes in blood flow or wall shear forces (1). This regulatory process, which acts to maintain constant wall shear (2-5), requires an intact endothelium (6;7). In experimental hypertension in animals, and in human essential hypertension, the presence of endothelial cell dysfunction (8;9) and elevated wall shear forces (10-13) suggest that this important regulatory mechanism is impaired. Similarly, in mature animals' shear-mediated changes in large artery structural diameter is attenuated (14;15) suggesting that shear-regulated processes may be altered with aging.
Based upon these observations, we conducted studies to investigate the whether alterations in collateral artery development occurred in our model (Figure 1) within hypertensive and mature animals.
Collateral Development and Hypertension
In Male Wistar-Kyoto (WKY) and spontaneously hypertensive rats (SHR) a collateral dependent region was created within the intestinal vasculature. Diameter measurements of both normal (control) and collateral vessels were made at the time of model creation. Flow and diameter measurements were made seven days post-ligation. Histological examination of the arteries occurred following the second measurement time point. In vivo diameter and wall areas were determined from videography, and tissue sections, respectively, using the Image-1 analysis system. The number of nuclei within the intima and media were counted manually. Wall shear rate was calculated from diameter and flow measurements using the formula: WSR = (4Q)/(Pr3) where Q is blood flow (ml/sec) and r is the vessel radius (cm).
Arterial wall shear rates were increased significantly in all collateral arteries (Figure 2). The shear rates in the arteries from the hypertensive rats were significantly higher than the shear rates in arteries from the normotensive animals. This suggests that shear-mediated regulation of arterial diameter is suppressed or that the set point for regulation is altered.
Figure 2. Average arterial wall shear rates presented in the table below.
Morphometric and histological data (Figures 3-6) indicate that the remodeling processes associated with luminal expansion are also abnormal in the SHR. The medial thickness to luminal radius relationship is preserved during luminal expansion of small arteries in normotensive(Unthank et al., 1996a) but not hypertensive animals (data not shown). Arteries in the SHR appear to have a greater number of endothelial cells than normotensive arteries initially (Figure 5) but the degree of endothelial proliferation during shear-mediated growth(Unthank et al., 1996a) is suppressed in the hypertensive animals.
Figure 3. Within each animal, the growth of the collateral arteries was compared to the growth of normal arteries from the diameter measurements made prior to and one-week after creation of the model.
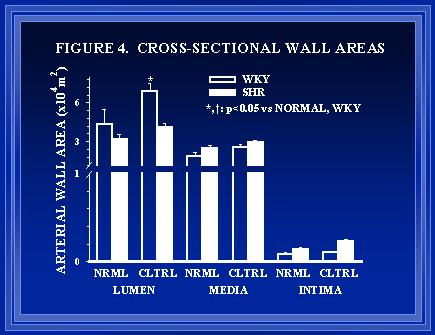
Figure 4. Cross-sectional areas of the lumen, media, and intima of normal (NRML) and collateral (CLTRL) arteries in WKY and SHR are shown. Consistent with the in vivo diameter measurements of Figure 3, luminal area is much greater in collaterals of WKY than SHR. Although medial area was not significantly greater in SHR than WKY arteries, the medial thickness was greater in SHR for both normal (34±2 vs 22±2 µm) and collateral arteries (35±2 vs 25±2 µm).
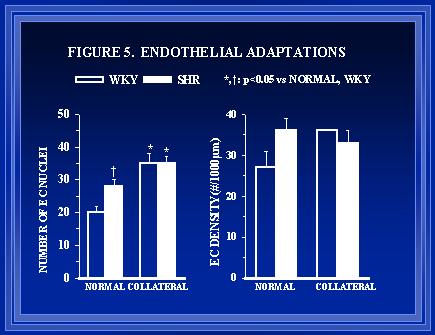
Figure 5. The total number of endothelial cell (E.C.) nuclei in arterial cross-sections and the EC density are shown in the left and right panels, respectively. The normal arteries in the SHR had a greater number of endothelial cells than WKY. Endothelial proliferation occurs in the collaterals of both groups, but the relative increase is less in the SHR than WKY.
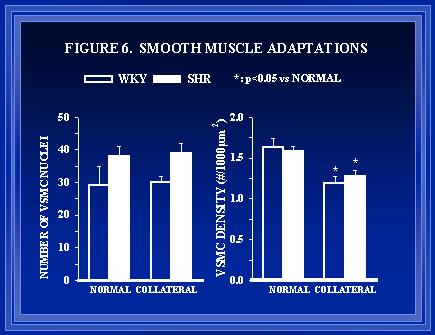
Figure 6. The total number of vascular smooth muscle cell (VSMC) nuclei in arterial cross-sections and the VSMC density (nuclei per 1000 µm2 of medial area) are shown in the left and right panels respectively. As luminal expansion occurs in collaterals of both WKY and SHR, the VSMC density is decreased.
Back to the top.
Collateral Development and Aging
Male Wistar rats, weighing ~200 g, and >500 g were used for the young (control) and aged (experimental) groups, respectively. Ligation of vessels to form the collateral pathway was performed as previously described (Unthank et al., 1996b; Unthank et al., 1996a) and illustrated in Figure 1. Measurements of both control and collateral vessel diameters were performed using in vivo microscopy under dilated conditions. One week later, measurements of these vessels were repeated again under dilated conditions. Histological and morphometric measurements of nuclear counts and wall areas were determined.
In young rats, significant shear-mediated luminal expansion occurred in the collateral vessels (Figure 7). This luminal expansion was associated with intimal hyperplasia (Figure 8) and medial hypertrophy (Figure 9). In older animals, intimal hyperplasia (Figure 8) and medial hypertrophy (Figure 9) were observed in the vessels subjected to elevated flow, however, significant luminal expansion did not occur within the one-week period (Figure 7).
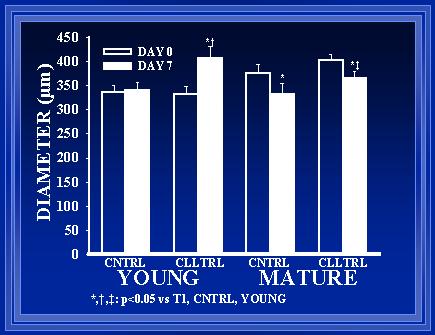
Figure 7 demonstrates that there was a significant increase in arterial diameter in the young rat collateral from time T1 until T2 (p<0.01). By chance, the collaterals in the mature rats were large at T1, but after one week, these vessels were significantly smaller at T2 (p<0.05). There was no significant difference between the normal vessels of the young rats from T1 to T2 (p<0.05).
Figure 8. A comparison of arterial diameter before and one-week after collateral pathway formation.
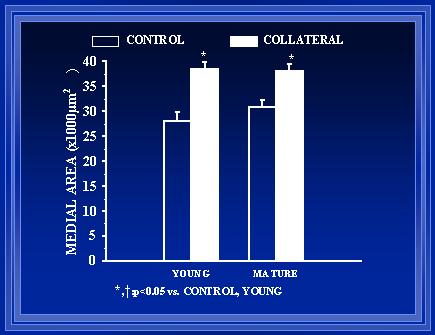
Figure 9. Endothelial cell counts from arterial cross sections reveal a significant difference after one week between young rats and aged rats (p<0.05). Also, when compared to the normal vessel of each rat, there is a significant increase in the endothelial cell counts of the collateral vessel compared to the normal vessel.
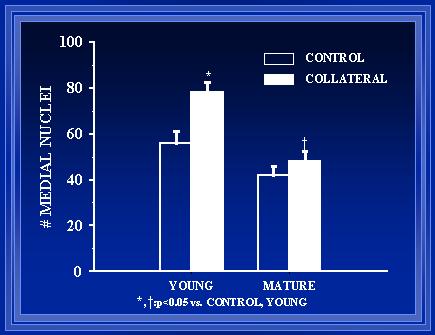
Figure 10. Collateral arteries in the aged rats were found to have a significantly greater medial area than the collateral arteries in the young rats at T2 (p<0.05). Of note, there is not a significant difference in the collateral artery diameters at T2 (refer to Fig. 3).
Our study of shear-mediated regulation of structural diameter in small resistance arteries of mature animals is similar to previous studies in large arteries which have demonstrated that diameter changes either do not occur(Miyashiro et al., 1997) or that the magnitude of the change is diminished(Langille et al., 1989). However, our study demonstrates that, in small arteries of mature animals, shear forces influence wall composition.
Back to the top.