April 25, 2024
McMaster students, community members to attend Tom Wilson’s musical ‘Beautiful Scars’
Read More
Share
SHARE WITH YOUR FRIENDS
Pick one or more destinations:
0
0
0
×
April 23, 2024
Student spends months getting covered in bird poop and vomit on uninhabited island — and can’t wait to go back
Read More
Share
SHARE WITH YOUR FRIENDS
Pick one or more destinations:
0
0
0
×
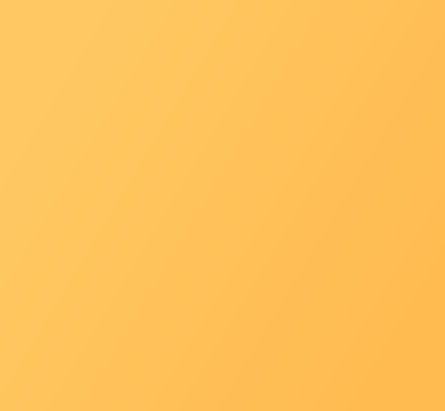
Humanities researchers working at the intersection of social challenges and the environment
Read More
Share
SHARE WITH YOUR FRIENDS
Pick one or more destinations:
0
0
0
×
April 23, 2024
Curator Q&A: Reframing the life of Lucy Catherine Russell
Read More
Share
SHARE WITH YOUR FRIENDS
Pick one or more destinations:
0
0
0
×
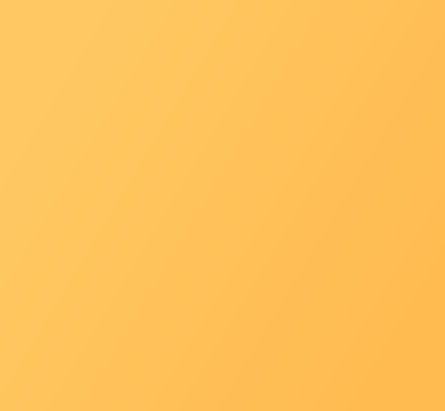
Analysis: New electrochemical technology could de-acidify the oceans – and even remove carbon dioxide in the process
Read More
Share
SHARE WITH YOUR FRIENDS
Pick one or more destinations:
0
0
0
×
April 22, 2024
Student brings Hamilton war hero’s history to life
Read More
Share
SHARE WITH YOUR FRIENDS
Pick one or more destinations:
0
0
0
×
April 22, 2024
So much more than a footpath: McMaster graduate on the importance of the Bruce Trail
Read More
Share
SHARE WITH YOUR FRIENDS
Pick one or more destinations:
0
0
0
×